Porites lutea
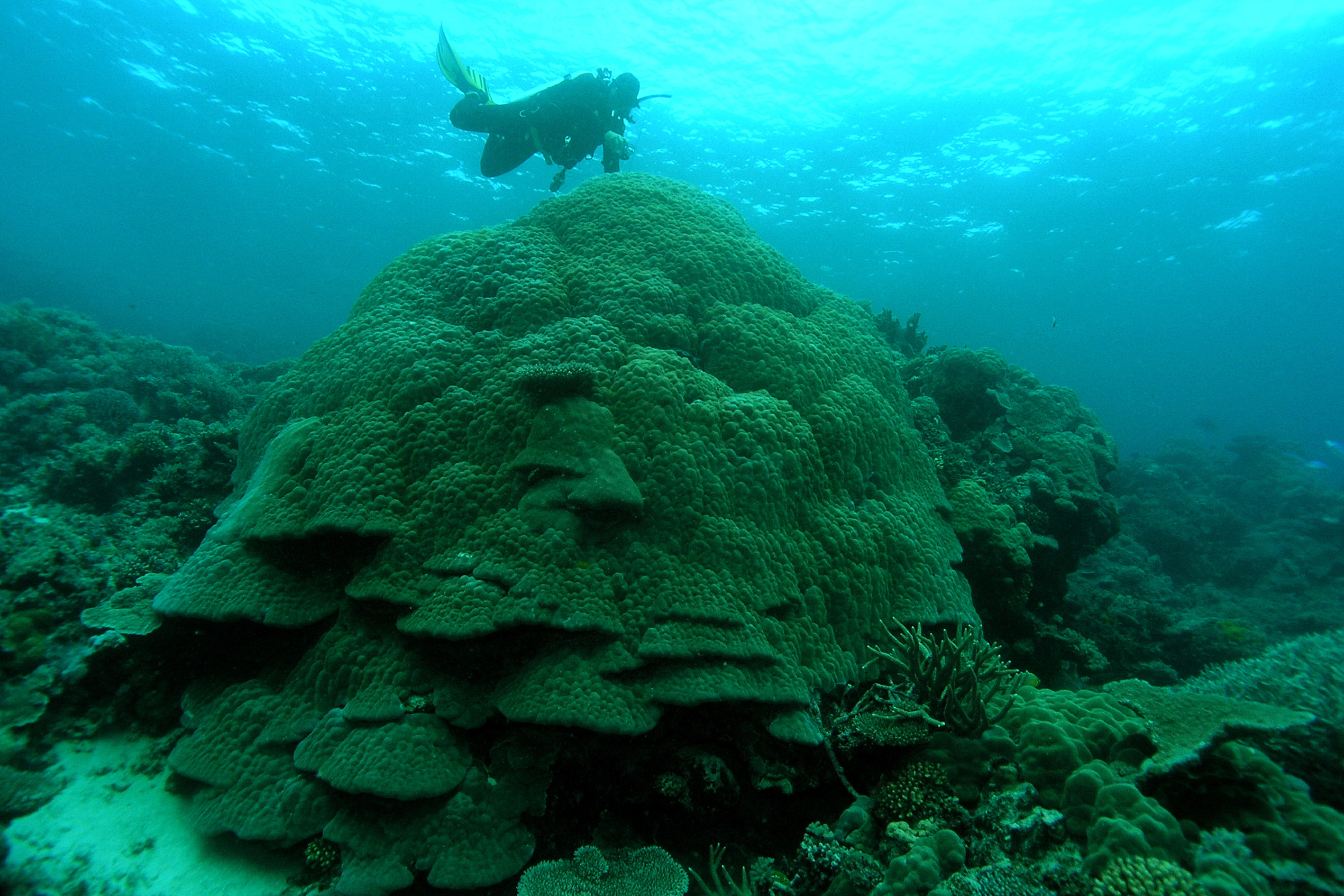
Figure 1.1. Porites sp. Scaled against a diver, showing the massive size that it can grow into, displaying its significance as a reef-building species. Copyright © 2008 Eric Matson
1 Introduction
Porites lutea Edwards & Haime, 1851 (Figure 1.1, 1.2) is hard coral from the family Poritidae that can be easily found around the tropical reefs of the world [1, 2]. They are practically impossible to identify to the species level without close examination and has been described as “large boulder-shaped (massive) colonies” among commonly seen hard corals for the general public [1]. It can only be identified to the species level after examining the corallite under a stereo microscope, using distinguishing features such as septa arrangements and presence of fusions (see Section 5.2 Morphology for details) [2, 3, 4]. Its name means yellow pore, based on the pore-like corallites of the genus and the yellow hue of the first specimen described [2, 5]. It is a rather common species of hard corals that can be found around Singapore, especially along shallow areas around the southern islands.
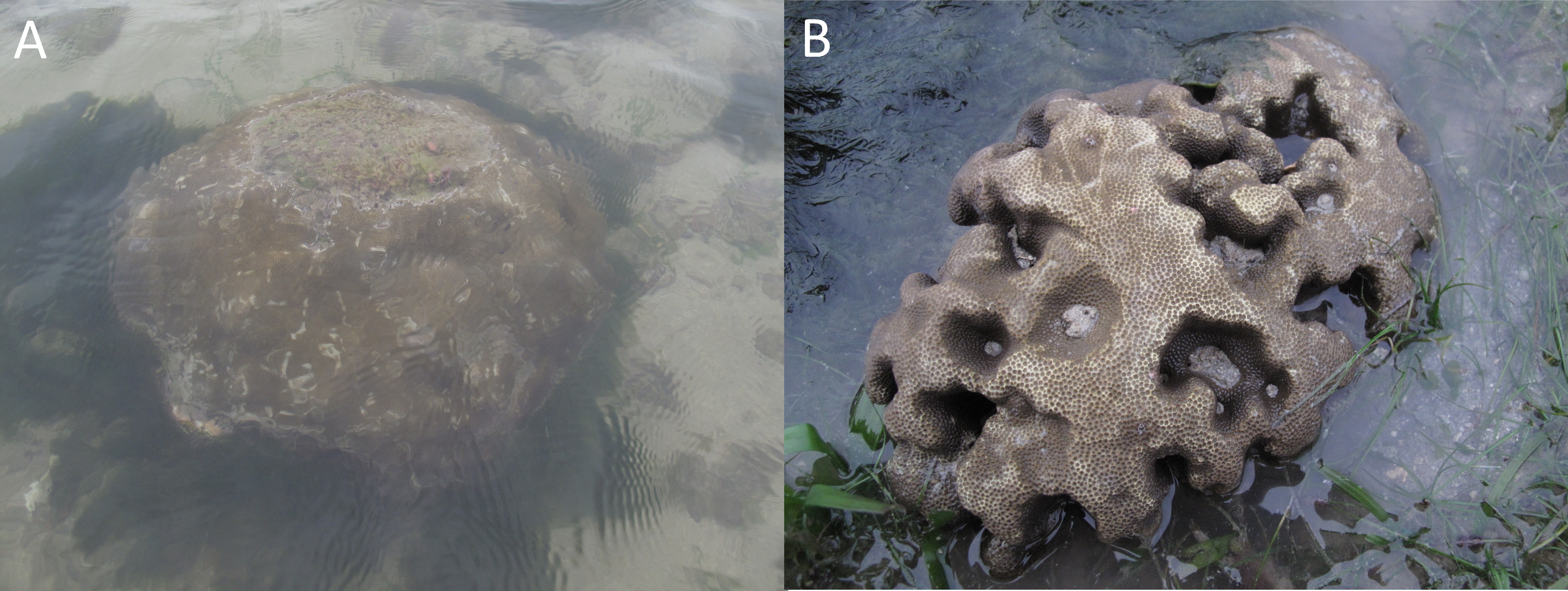
|
Figure 1.2. Porites lutea found in Cyrene Reef, Singapore. A shows a specimen submerged in shallow waters, while B shows a specimen half exposed and also with a strange shape.
|
Like other Porites, it is an important reef-building species due to its ability to grow to immense sizes (Figure 1.1) [2, 5], providing shelter for other animals in the reef system. It is also an extremely hardy and sturdy genus, allowing them to weather well against storms and strong waves, increasing their importance as reef-building species.
Porites lutea also exhibits high levels of halotolerance, tolerating daily tidal shifts of 10 – 30% salinity (Figure 1.2) [6]. When salinity declines, photosynthesis rates decline as the corals contracts it polyps to protect them. They then maintain their metabolism through consuming prey, such as brine shrimp and zooplankton [6].
2 Distribution
2.1.1 Habitat
It occurs commonly with other Porites species, growing on the back of reef margins, fringing reefs and lagoons [2]. It is also common to observe them in intertidal zones exposed during low tide, they are known to survive well through durations of exposure [1, 2, 3].
2.1.2 Local Distribution
Locally, it is commonly found along remaining coral reefs and shores during low tide. A few notable areas where it can be found includes Raffles Lighthouse, Pulau Semakau, Pulau Jong, Sisters Island, Pulau Hantu, St. John’s Island, Kusu Island and Cyrene Reefs [3, 4]. Additionally, unconfirmed sightings also possibly place these species along the northern shores [1]. The P. lutea and few similar species of Porites such as P. lobate and P. australiansis can be easily distinguished based on their moderately large size and pore-like surface (Figure 1.2), though identifying them by species will not be possible.
Map 1. Showing local areas where P. lutea can be found [3, 4]. Adapted from Google Maps.
2.1.3 World Distribution
The coral inhabits reefs across the tropics, stretching from the Red Sea, Western Indian Ocean to the French Polynesian Islands [2, 5].
Map 2. Geographical distribution of P. lutea, with occurrences marked in dull yellow. The map is adapted from IUCN.
3 Biology
3.1 Coral anatomy
Basic coral anatomy can be seen in Figure 3. Most of the identifying features of the genus Porites can be seen from the calice. Key differences between species are from septa arrangements, presence and structure of paliform lobes (or pali) and structure of the columella. These are important keywords that will be used throughout parts dealing with morphology.
Figure 3. Basic anatomy of corals, with labels of key characters that are used through this page. Copyright © 2000 Veron
The general structure and coral anatomy can be seen from Figure 3. The massive structures we usually see, as observed from Figure 1, are mostly made up of the skeleton. The living part of the coral is usually only at the surface which contains the polyp.
3.2 Growth
Coral grow by secreting a skeleton of calcium carbonate (CaCO3) below their polys. The shape may vary depending on species and environmental factors, but P. lutea mainly takes on the massive shape [7]. Massive corals are bulbous in shape and rounded, they may range from smaller 50 cm in diameter colonies, to a larger 5 m in diameter (Figure 1.1) [2, 5]. Growth speed of P. lutea has been measured to about 10.3 mm per year or 2.4 g per cm2 per year [7, 8, 9]. Porites are generally not exceptionally fast growers and are known to have declining growth over recent decades with the increased sea temperature due to global warming [8].
3.3 Feeding
Corals feed using two ways, as seen in Video 1. The main method which they feed, and where they get most of their energy from is through a symbiotic relationship with zooxanthellae. The corals provide a home and shelter these zooxanthellae, whom in return, provide glucose, glycerol and amino acids through photosynthesis for the coral [10, 11]. The coral uses these to produce proteins, fats, carbohydrates and calcium carbonates which form the skeleton [12, 13, 14, 15, 16].
Video 1. Feeding process of corals. (Copyright
© 2015 Khaled bin Sultan Living Oceans Foundation)
The second method is through food capture using their tentacles which has nematocysts [10, 11]. This usually occurs at night. Nematocysts are cells that contain a secretory organelle containing toxins which are used for prey capture and defence [17], they are able to fire upon contact and pierce through soft tissue [17], as seen in Video 2.
Video 2. Stinging cells of nematocysts in slow motion. (Copyright
© 2014 Smart Everyday)
3.4 Reproduction
Corals are able to reproduce both asexually and sexually. Asexual reproduction occurs through the budding of colonial polyps from the parent polyps [16]. This usually occurs only after the main colony or parent polyp grows to a certain size, after which, it is a continual process throughout the polyp’s life [13].
For stony corals or hard corals, most are broadcast spawners, like P. lutea. This means they release a large number of sperm and eggs into the water to spread their young over broad areas [2]. They then form free-floating larvae known as planulae. This mass spawning is timed using environmental triggers such as lunar cues for short-term control, and temperature, day length and/or temperature change for long-term control [2, 13]. Long-term controls define the period or season in which spawning may occur, usually around the months of April, while short-term controls define the exact moment or day in which the spawning actually occurs. The mass spawning can be seen in Video 3.
Video 3. Mass spawning of corals. (Copyright © 2017 Ocean Today)
4 Ecology
The ecology of P. lutea is a broad one. Its importance includes the species itself, as a home for several parasitic species of borers, the genus as important reef builders, and corals as an entire reef system. Because of its multi-level importance, the threats that place the corals at risk should be looked at in earnest. The value of coral reefs as a whole is not something that can be replaced.
4.1 Importance of Corals and Reef systems
4.1.1 Reef-building
Reef-building corals or hermatypic corals are corals in the order Scleractinia that build reefs through the calcium carbonate they secrete [18]. The deposited calcareous material form a stony framework which becomes a reef. Corals that are unable to secrete hard calcareous material are referred to as ahermatypic or non-reef-building species [18]. As such, the core of every coral reef is their hermatypic corals. Porites lutea is an important species among hermatypic corals. Its shows resilience in shallow waters, an ability to grow to immense size and a growth form that shelters the reef from storms and waves [2, 5, 9]. This allows it to be a persistent resident of the reef and a significant contribution to its mass [9].
4.1.2 Coral reefs
Coral reefs are among the most diverse and valuable ecosystems on Earth, housing thousands of vertebrate species and hundreds of coral species with an estimated 1 – 8 million undiscovered species [19]. Reefs also hold immense potential for wealth. Providing both economic and environmental services to many people, the goods and services are worth $375 billion each year [20]. Coral reefs also provide physical structure, social/cultural, biotic, biogeochemical and information services, giving them immense value, especially for coastal dwellers [21]. They also provide important nurseries for fishes and some riverine organisms [22].
4.2 Threats to Corals and Reef systems
4.2.1 Natural Threats
Porites lutea are known to inhabit shallow waters [2, 3, 4, 5], and as such are at risk of tidal emersions. Long periods of low tides leave coral heads exposed in shallow waters, damaging the reefs. This may cause physiological stress which may result in the expulsion of their symbiotic zooxanthellae, this may end in death [13]. Shallow reefs also mean greater exposure to weather conditions like hurricanes, cyclones and powerful waves. These may smash against coral heads, causing fragmentation and damage [13, 23]. Slow growing corals are particularly vulnerable as the long recovery time may lead to algal overgrowth [24]. Porites lutea is also exposed to similar risk, but are less vulnerable to these threats as they are large massive corals able to take punishment from storms and waves. They are also halotolerant, meaning able to tolerate flux in salinity well.
Natural predation from fish, marine worms, barnacles, crabs, snails and sea stars all prey on the soft inner tissues of coral polyps [23]. Particularly damaging incidences of predation include outbreaks of crown-of-thorns starfish, Acanthaster planci [25, 26]. These outbreaks are severe and cause mass mortality of corals, resulting in cascading effects onto entire reef ecosystems [27].
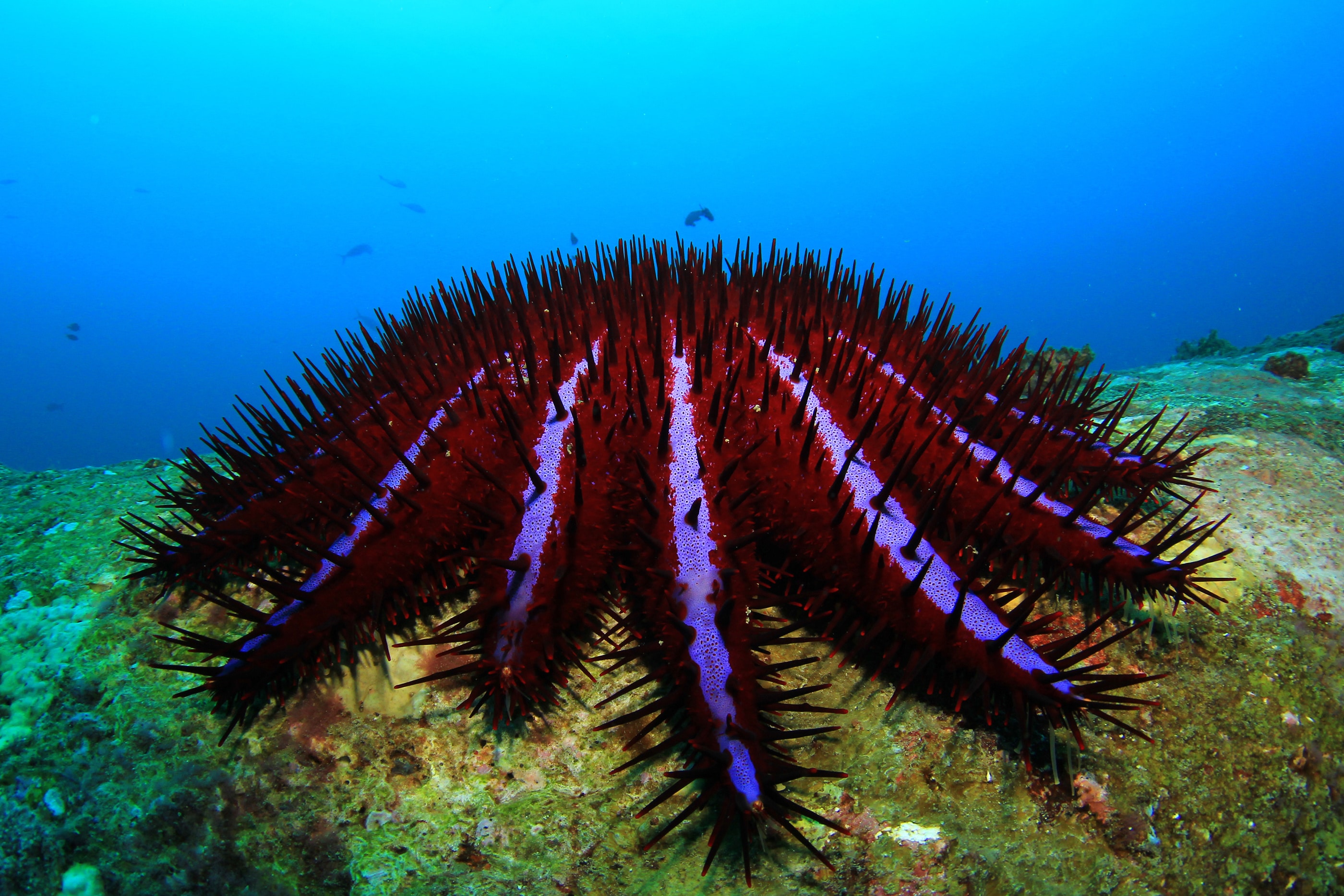
Figure 4. Crown-of-thorns starfish. (Copyright
© Charles Davis)
4.2.2 Threat of climate change
Large-scale changes such as rising sea surface temperature, salinity changes due to El Nino and ocean acidification are one of the many natural phenomena afflicted our oceans today. The combination of factors may lead to disastrous effects on a coral’s physiology, causing severe and extensive bleaching of the corals, eventually leading to their death [28]. The changes may also lead to growth declines and an overall drop in coral health [8].
4.2.3 Conservation in Singapore
Singapore, though small, does extensive research in corals. Being situated near the coral triangle, it is a prime location for research. The Tropical Marine Science Institute (TMSI) of Singapore is an institute formed within the National University of Singapore, they have done numerous activities with regards to conservation. They have done conservation-related research, especially in building coral nurseries to help build up coral reefs.
Seagrass-Watch is a global scientific, non-destructive, seagrass assessment and monitoring program that has a base here in Singapore. Though not focused on coral specifically, they organise volunteer work as well that benefits local coral reefs.
Hantu Divers is the only non-profit organization in Singapore that conducts regular guided dives for members of the public. They do educational tours and assist scientists with data collection and basic monitoring activities. They also regularly do volunteer work to help coral reefs around Singapore. They accept volunteers as well, visit here to learn more.
Wild Singapore is an independent online resource run and funded by Ria Tan. They also do organise small volunteer efforts to help marine conservation. The resources provided also include several corals, but are limited in details.
4.3 Associated Species
Associated species to P. lutea revolve mainly around the coral’s ability to provide a home for organisms, providing a refuge from predators in the coral reefs. Organisms that are best able to benefit from the commensalism are boring organisms, which are able to burrow into the hard skeleton of P. lutea. The most commonly associated species to P. lutea observed is a mutualistic pair of shrimp and shrimp goby [29]. Shrimp species of the genus Alpheus (Decapoda: Alpheidae)and goby species of the genus Ctenogobiops (Perciformes: Gobiidae)are often found burrowed into the skeleton of P. lutea [29]. Some specific species observed for Alpheus are A. mallaeodigitus and A. djeddensis [29, 30].
Other organisms observed are coral boring species from the genus Lithophaga (Bivalvia: Lithophaginae) [31] and a serpulid worm Spirobranchus giganteus (Canalipalpata: Serpulidae) [32]. Though not as prevalent as the mutualistic shrimp and goby, they are considerably widespread as well [31, 32].
4.4 Paleoclimatology
The genus Porites has been shown to be considerably good recorders of past marine surface conditions [33]. The aragonitic skeleton of specimens contains oxygen isotopic composition which corresponds with oxygen isotopic composition and temperature conditions of the seawater at the time of growth [34]. These relationships are important in the reconstruction of past climates and related global climate patterns such as the El-Nino Southern Oscillation.
5 Taxonomy
This section leans strongly towards extremely technical word use and forms of explanation, parts have been simplified as far as possible while maintaining its scientific integrity. Please refer to Coral anatomy for morphological jargon. This section is aimed at people who would require the technical knowledge on specific corals, identification and phylogeny. It was made as a brief summary of P. lutea taxonomy, both its morphological and phylogenetical aspects.
Morphology and phylogeny are used very different with respect to Poritidae because of a few inherent issues with the family. The main species concept being used is the typological species concept [2, 5]. Corals are mainly distinguished as different species based on their skeletal features, mainly how their corallites are structured, based on the original specimen described [2, 5]. Although other species concepts may be applied more efficiently, it remains that specimens are mainly identified to species level based on morphological similarities with type specimens. Phylogeny, on the other hand, is mainly used for deciphering the relationships between ‘identified’ species [35].
Specific to the genus Porites, there are major taxonomic controversies due to high colony variation and lack of distinct skeletal features. Though there are some identifying features between species, they remain ambiguous and may have variations between populations that make clear identification difficult [36]. Coding genes such as COI, ITS and COX1 are reputably unreliable to delimit species for corals and are especially bad for Porites [37, 38, 39]. It is because of these two main prevailing issues that make the taxonomic structure of Porites especially messy and difficult to clearly demarcate [40], though Kitano et al. [35] have made a remarkable attempt at building a phylogeny tree of the family Poritidae.
5.1 Scientific Classification
Kingdom:
|
Animalia
|
Phylum:
|
Cnidaria
|
Class:
|
Anthozoa
|
Order:
|
Scleractina
|
Family:
|
Poritidae
|
Genus:
|
Porites
|
Species:
|
P. lutea
|
5.2 Morphology
5.2.1 Original diagnosis & translation
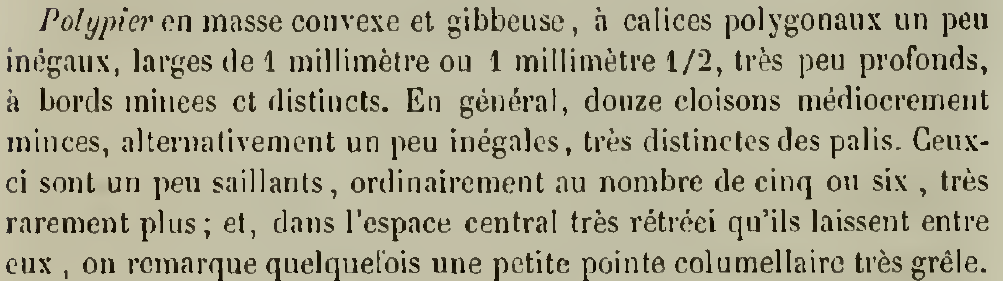
|
Polypier in convex and gibbous mass, with polygonal calices a little unequal, broad of 1 millimetre or 1 millimetre 1/2, very shallow, with distinct and distinct edges. In general, twelve poorly thin partitions, alternately a little unequal, very distinct from the pali. These are a little salient, usually five or six, very rarely more; and in the much narrowed central space which they leave between them, one sometimes notices a small, very small columella point.
|
Figure 5.1. Original description from Edwards & Haime [41]
|
Translated using Google translator
|
5.2.2 Holotype
The holotype can be found in the Muséum national d’Histoire naturelle de in Paris, France (MNHM); Z. 191a
A holotype is the original specimen used to describe the species. It is important taxonomically as it is used as the base in which a 'species' is decided upon among organisms of Scleractina. This gives rise to issues when genetic work was brought into the field of taxonomy as holotypes lacked any genetic material.
5.2.3 Description
(Based on samples collected [4])
Colonies hemispherical or helmet-shaped and large (Figure 1.2). Surface smooth and brown. Corallites small (1-1.5mm) with no coenosteum. Little to no skeletal variations between material examined. All had the distinctive shorter dorsal directive and triplets fused to form a trident (Figure 5.2.1 and 5.2.2). Walls short but form a ridge-like structure that forms a clear distinction between corallites, not present for all.
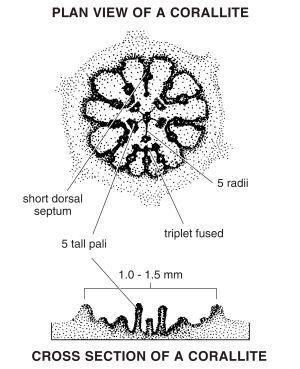
|
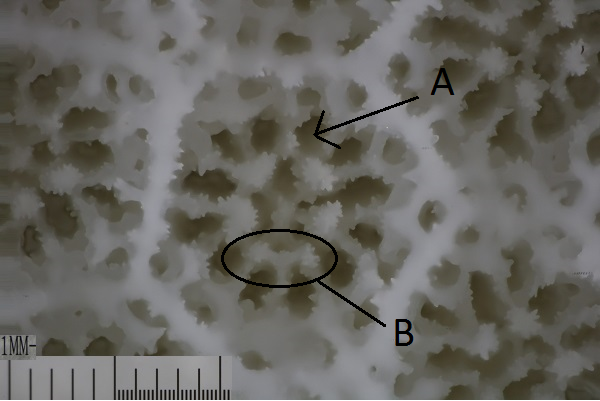
|
Figure 5.2.1. Illustration showing the identifying features of P. lutea using morphological differences in the calice. Copyright © 2000 Veron [2]
|
Figure 5.2.2. Image showing an actual calice of P. lutea. A points towards the distinctively shorter dorsal directive/septum, while B shows the fused triplets forming a trident shape.
|
5.2.4 Diagnosis
(Based on [4])
Colonies are massive (Figure 5.3.1) and form a hemispherical shape that grows to 2m. The surface is smooth with irregular humps across the colony. At the coral edge, thick ledges are seen forming, usually indicating the end of the living coral. Calices are small (1.5mm) and shallow (Figure 5.3.2). Calices have thin walls that are perforated, walls grow out to form ridge structures that clearly divide corallites. Dorsal directive is shorter than the rest and the ventral directive is fused to form a trident (Figure 5.3.3). The fused ventral directive that forms a trident may not be seen throughout the coralla and only forms at ends of septa. Five distinct pali, dorsal directive lacks one, the triplets formed one and the lateral pairs each have one. Columella is weakly developed and absent in some, columella forms a star shape connecting to 5 pali (Figure 5.3.3). Two synapticular rings are observed, one outer and other palar.
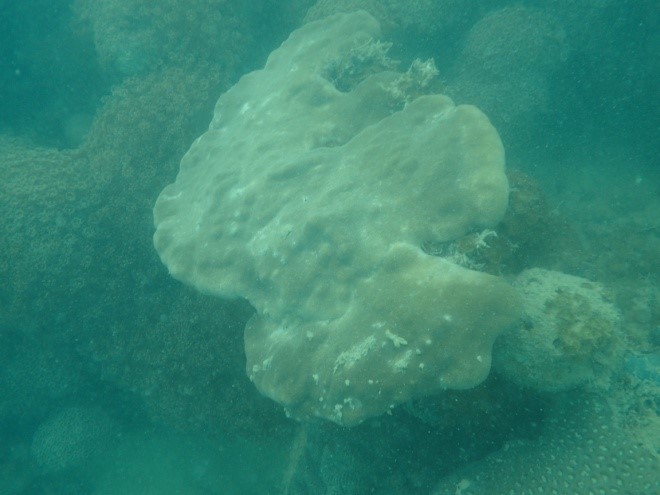
|
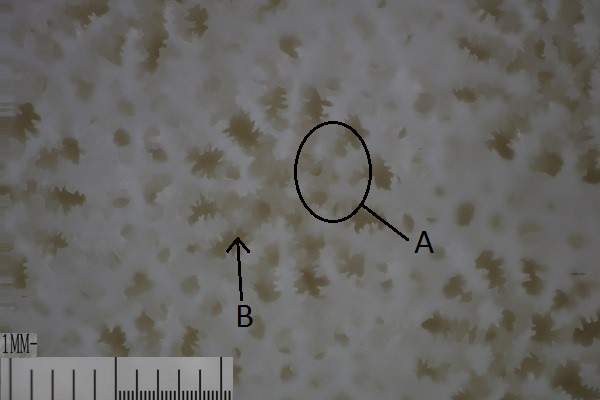
|
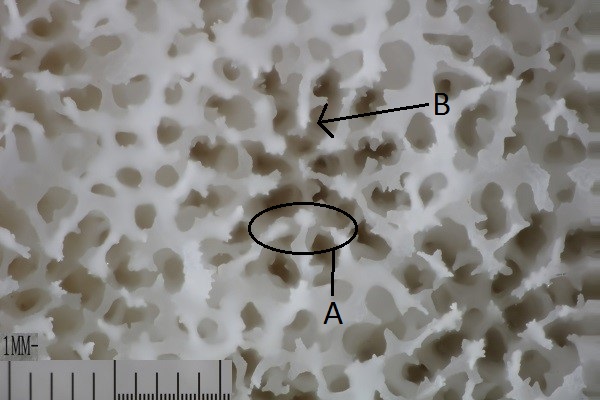
|
Figure 5.3.1. Lobe form of P. lutea, showing its massive growth structure
|
Figure 5.3.2. Corallite of P. lutea where A shows the fused triplets forming a trident and B shows the distinctively shorter dorsal directive/septum.
|
Figure 5.3.3. Corallite of P. lutea where A shows the fused triplets forming a trident and B shows the distinctively shorter dorsal directive/septum.
|
5.2.5 Similar species
Although Porites can be identified down to species level using a stereo microscope, there are several extremely similar species that complicates identification [2, 5]. Though the similarity between species of the genus is subjective, a comparison is drawn up here to better outline the difficulty in identifying species of the genus Porites.
Identifying features between P. lutea and P. lobata may seem distinct enough in illustration (Figure 5.4.1 and 5.4.2). Clear differences in triplet fusion and a larger difference in the centre, where P. lutea has a distinguishing star in its columella. Porites lobata, on the other hand, lacks these traits.
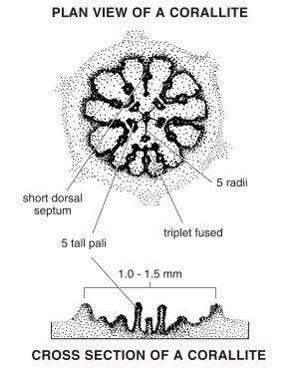
|
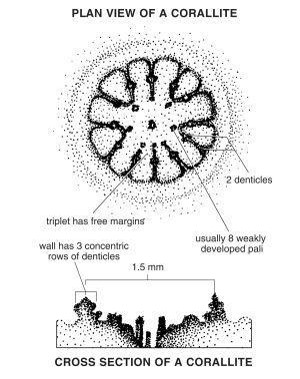
|
Figure 5.4.1. Illustration showing the identifying features of P. lutea using morphological differences in the calice. Copyright © 2000 Veron [2]
|
Figure 5.4.2. Illustration showing the identifying features of P. lobata using morphological differences in the calice. Copyright © 2000 Veron [2]
|
When examining the actual corallite itself, many corallites are damage or have small minor abnormalities. Distinguishing features for P. lutea in Figure 5.4.3 were no clear. Triplet fusion seemed ambiguous and semi-formed, though the star shape for its columella seems clear enough. Figure 5.4.4 shows P. lobata with not so distinct space between triplets, excessive dentation makes distinguishing absence of a star-shaped columella hard as well.
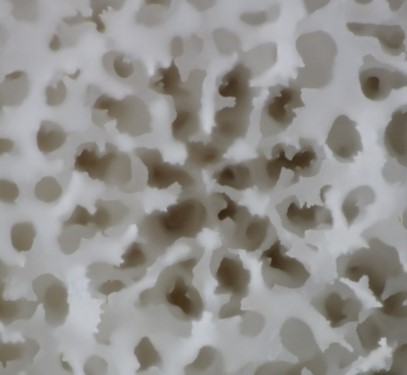
|
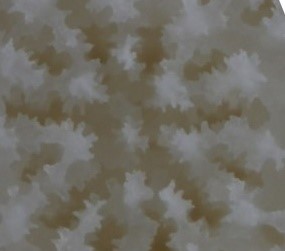
|
Figure 5.4.3. Close up of P. lutea calice, the fusion of triplets are not as clear with the star in the centre not too distinct. Certain corallites may not be well developed or have abnormal features, leading to misidentification.
|
Figure 5.4.4. Close up of P. lobata calice, older corallites like this one tend to have extreme dentation, “fatter” more calcified calice. Makes distinguishing features harder; triplets separation not clear, lack of star in the back not distinct.
|
Non-corallites features such as shape, size and colour of the coral colony are completely unable to help at all. As seen in Figure 5.4.5 and 5.4.6, they have almost no known differences between each other. Corals also tend to show high levels of plasticity and may change significantly depending on environmental factors.
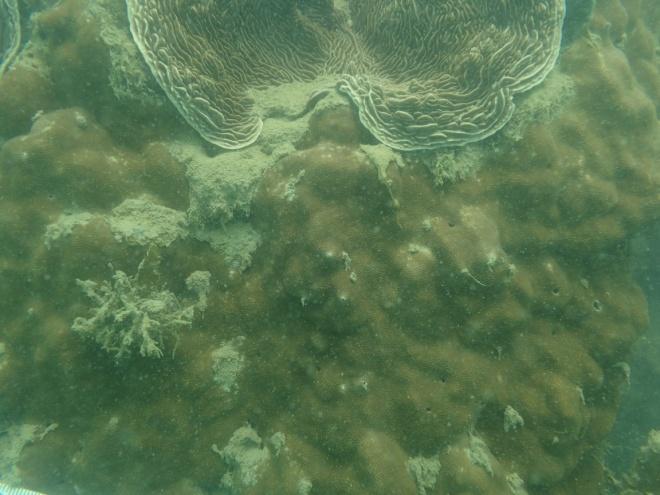
|
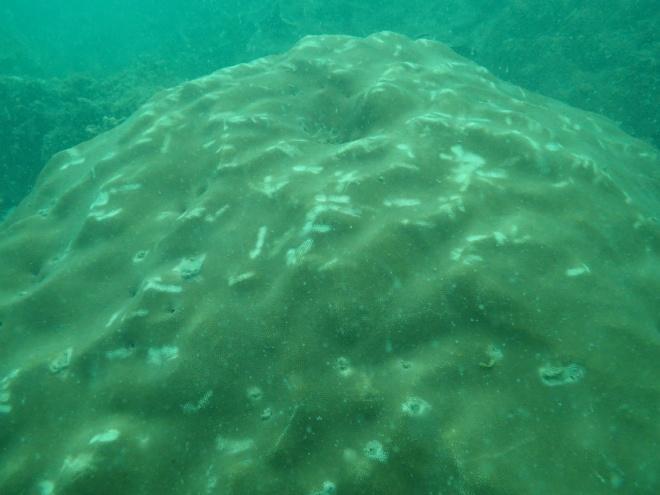
|
Figure 5.4.5. Massive coral colony, later identified as P. lutea.
|
Figure 5.4.6. Massive coral colony, later identified as P. lobata.
|
5.3 Phylogeny
Kitano et al. [35] recent paper was the only attempt at looking to the phylogeny of Poritidae (Figure 5.5). The paper revealed interesting relationships between several genera of corals, including several taxonomical shifts [40, 42]. Even with all this new finding, however, support for species delimitation is not clear [35]. A major recent shift is the transfer of the genus Alveopora to Acroporidae [42]. Though morphologically, there were subtle differences between Alveopora and the other genera of Poritidae, particularly septal fusion present in Porites and Goniopora [5], the distinction on a genetic level was what led to its shift [40].
Porites lutea is settled very strongly within the genus as seen in Figure 6, though a few new members were added in [35, 42]. It remains, however, that species identification is still heavily depended on morphological features [2, 5, 35, 40, 42].
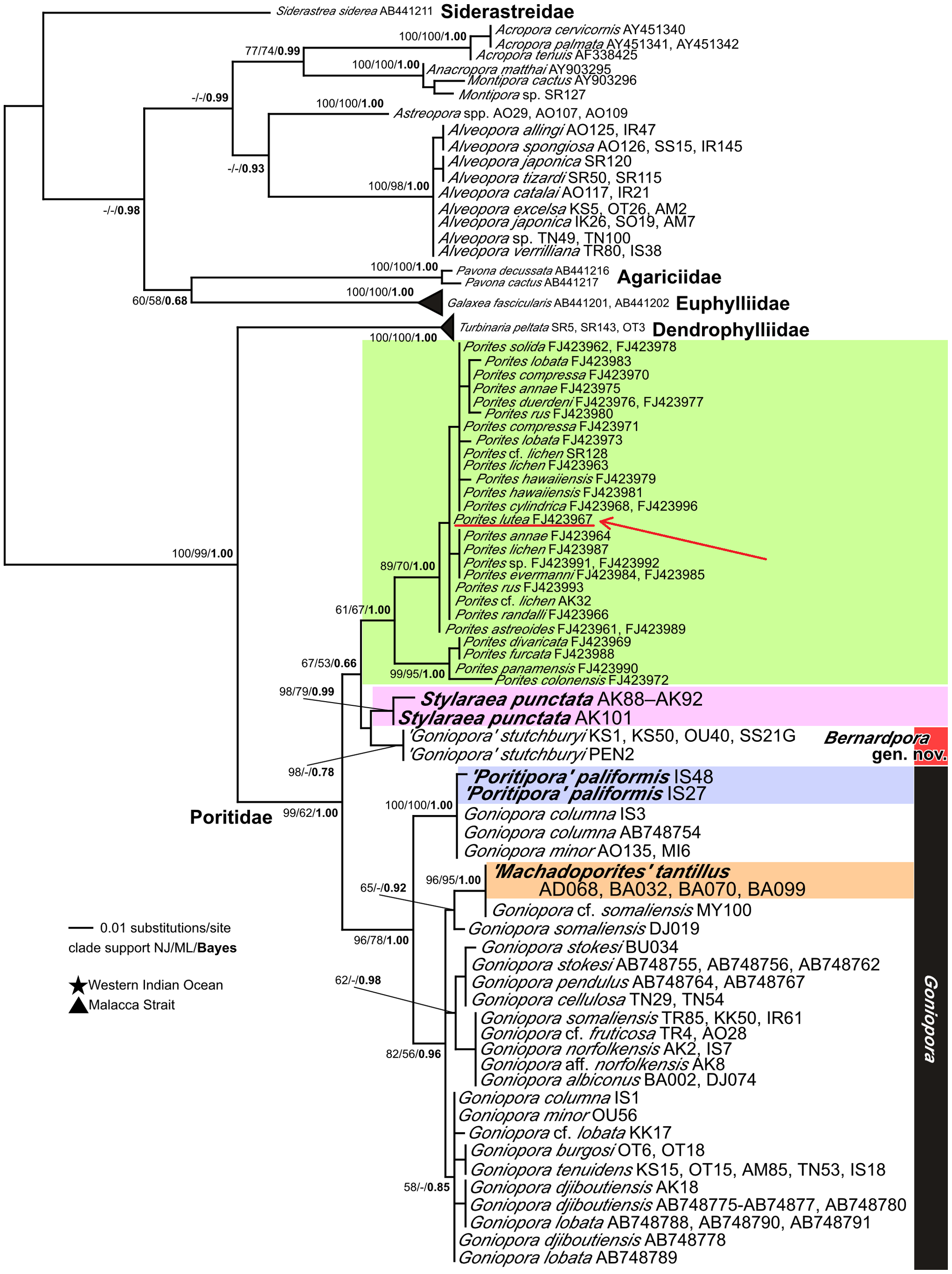
|
Figure 5.5. Phylogeny tree from Kitano et al. [35], P. lutea is underlined and pointed out in red.
Kitano et al. original figure description:
Molecular phylogenetic relationships of the family Poritidae and related families based on mitochondrial COI sequences. Numbers on/below main branches show bootstrap values (.50%) in ML and NJ analyses, and Bayesian posterior probability (.0.8). Stars show specimens collected from the western Indian Ocean, and triangles show ones collected from Malacca Strait.
|
5.3.1 Methods for Phylogeny Tree
The phylogeny tree was made by the following method:
- DNA sequence alignment was done using MAFFT 7 using L-INS-i option. Indels and alignment ambiguities were excluded manually.
- MEGA 4.0.2 was used to calculate pairwise genetic distances as p-distance.
- Phylogenetic trees were reconstructed using neighbour-joining (NJ) and maximum likelihood (ML).
- For NJ, PAUP 4.0b10 option was used to infer topologies for both COI and ITS markers using Kimura 2-parameter model and a bootstrap analysis.
- For ML, nucleotide evolution was assumed and assessed using Akaike's Information Criterion (AIC) as per MrModeltest 2.2.
- Genetic Algorithm for Rapid Likelihood Inference (GARLI) was used to find optimal ML topologies using default settings and models selected by MrModeltest 2.2; bootstrap analyses (500 replicates) was done for each marker.
- An initial quarter of the total phylogeny trees were rejected out of the trees that were sampled every 100 generations as burn-in. Remaining trees were then used to produce a 50% majority rule consensus tree.
6 References
- Ria, T. (2008). Porites. Retrieved from http://www.wildsingapore.com/wildfacts/cnidaria/coralhard/poritidae/porites.html
- Veron, J. E. N. & Pichon (2000). Corals of the World. Townsville: Australian Institute of Marine Science.
- Pang, S. & Huang, D. (n.d). Morphological taxonomy of Poritidae corals in Singapore.
- Huang, D., Tun, K. P., Chou, L. M., & Todd, P. A. (2009). An inventory of zooxanthellate scleractinian corals in Singapore, including 33 new records. Raffles Bulletin of Zoology, 22, 69-80.
- Veron, J. E. N. (1986). Corals of Australia and the Indo-Pacific. Sydney: Angus & Robertson
- Moberg, F., Nyström, M., Kautsky, N., Tedengren, M., & Jarayabhand, P. (1997). Effects of reduced salinity on the rates of photosynthesis and respiration in the hermatypic corals Porites lutea and Pocillopora damicornis. Marine Ecology Progress Series, 53-59.
Scoffin, T. P., Tudhope, A. W., Brown, B. E., Chansang, H., & Cheeney, R. F. (1992). Patterns and possible environmental controls of skeletogenesis of Porites lutea, South Thailand. Coral Reefs, 11(1), 1-11.- Tanzil, J. T. I., Brown, B. E., Tudhope, A. W., & Dunne, R. P. (2009). Decline in skeletal growth of the coral Porites lutea from the Andaman Sea, South Thailand between 1984 and 2005. Coral reefs, 28(2), 519-528.
- Potts, D. C., Done, T. J., Isdale, P. J., & Fisk, D. A. (1985). Dominance of a coral community by the genus Porites (Scleractinia). Marine Ecology Progress Series, 79-84.
- National Ocean Service (2017). Corals get their food from algae living in their tissues or by capturing and digesting prey. Retrieved from https://floridakeys.noaa.gov/corals/coralseat.html
- SeaWorld Parks & Entertainment (2017). Corals & Coral Reefs. Retrieved from https://seaworld.org/en/animal-info/animal-infobooks/coral-and-coral-reefs/diet-and-eating-habits
- Barnes, R. D. (1987). Invertebrate Zoology; Fifth Edition. Fort Worth, TX: Harcourt Brace Jovanovich College Publishers.
- Barnes, R. S. K., & Hughes, R. N. (1999). An Introduction to Marine Ecology; Third edition. Oxford, UK: Blackwell Science Ltd. pp. 117-141.
- Lalli, C. M., & Parsons T. R. (1995). Biological Oceanography: An Introduction. Oxford, UK: Butterworth-Heinemann Ltd. pp. 220-233.
- Levinton, J. S. (1995). Marine Biology: Function, Biodiversity, Ecology. New York: Oxford University Press, Inc. pp. 306-319.
- Sumich, J. L. (1996). An Introduction to the Biology of Marine Life, sixth edition. Dubuque, IA: Wm. C. Brown. pp. 255-269.
- Kass-Simon G. & Scappaticci A. A. (2002). The behavioral and developmental physiology of nematocysts. Canadian Journal of Zoology. 80: 1772 - 1794.
- Schuhmacher, H., & Zibrowius, H. (1985). What is hermatypic?. Coral reefs, 4(1), 1-9.
- Reaka-Kudla, M. (1996). The global biodiversity of coral reefs: a comparison with rain forests. In: D.E. Wilson, E.O. Wilson (eds.), Biodiversity II: Understanding and Protecting our Biological Resources. Washington, D.C.: Joseph Henry Press. pp. 83-108.
- Costanza, R., d'Arge, R., De Groot, R., Farber, S., Grasso, M., Hannon, B., ... & Raskin, R. G. (1997). The value of the world's ecosystem services and natural capital. nature, 387(6630), 253-260.
- Moberg, F., & Folke, C. (1999). Ecological goods and services of coral reef ecosystems. Ecological economics, 29(2), 215-233.
- Nagelkerken, I., Van der Velde, G., Gorissen, M. W., Meijer, G. J., Van't Hof, T., & Den Hartog, C. (2000). Importance of mangroves, seagrass beds and the shallow coral reef as a nursery for important coral reef fishes, using a visual census technique. Estuarine, coastal and shelf science, 51(1), 31-44.
- Jones, O. and R. Endean. 1976. Biology and Geology of Coral Reefs, vols. 2 & 3. New York: Academic Press Inc. pp. 216-250.
- University of the Virgin Islands (UVI) (2001). Threats to Coral Reefs.
- Moran, P. J., Bradbury, R. H., & Reichelt, R. E. (1988). Distribution of recent outbreaks of the crown-of-thorns starfish (Acanthaster planci) along the Great Barrier Reef: 1985–1986. Coral Reefs, 7(3), 125-137.
- Moran, P. J., De'ath, G., Baker, V. J., Bass, D. K., Christie, C. A., Miller, I. R., ... & Thompson, A. A. (1992). Pattern of outbreaks of crown-of-thorns starfish (Acanthaster planci L.) along the Great Barrier Reef since 1966. Marine and Freshwater Research, 43(3), 555-567.
- Kayal, M., Vercelloni, J., De Loma, T. L., Bosserelle, P., Chancerelle, Y., Geoffroy, S., ... & Adjeroud, M. (2012). Predator crown-of-thorns starfish (Acanthaster planci) outbreak, mass mortality of corals, and cascading effects on reef fish and benthic communities. PloS one, 7(10), e47363.
- Forrester, A. L. (1997). The effects of El Niño on marine life. Cambridge Scientific Abstracts.
- Thompson, A. R. (2004). Habitat and mutualism affect the distribution and abundance of a shrimp-associated goby. Marine and Freshwater Research, 55(1), 105-113.
- Kropp, R. K. (1987). Descriptions of some endolithic habitats for snapping shrimp (Alpheidae) in Micronesia. Bulletin of Marine Science, 41(2), 204-213.
- Morton, B., & Scott, P. J. B. (1980). Morphological and functional specializations of the shell, musculature and pallial glands in the Lithophaginae (Mollusca: Bivalvia). Journal of Zoology, 192(2), 179-203.
- DeVantier, L. M., Reichelt, R. E., & Bradbury, R. H. (1986). Does Spirobranchus giganteus protect host Porites from predation by Acanthaster planci: predator pressure as a mechanism of coevolution?. Marine Ecology Progress Series, 307-310.
- Lough, J. M. (2010). Climate records from corals. Wiley Interdisciplinary Reviews: Climate Change, 1(3), 318-331.
- Thompson, D. M., Ault, T. R., Evans, M. N., Cole, J. E., & Emile‐Geay, J. (2011). Comparison of observed and simulated tropical climate trends using a forward model of coral δ 18O. Geophysical Research Letters, 38(14).
- Kitano, Y. F., Benzoni, F., Arrigoni, R., Shirayama, Y., Wallace, C. C., & Fukami, H. (2014). A phylogeny of the family Poritidae (Cnidaria, Scleractinia) based on molecular and morphological analyses. PLoS One, 9(5), e98406.
- Weil, E. (1992). Genetic and morphological variation in Caribbean and eastern Pacific Porites (Anthozoa, Scleractinia). Preliminary results. In Proc 7th int coral Reef Symp (Vol. 2, pp. 643-656).
- Shearer, T. L., Van Oppen, M. J. H., Romano, S. L., & Wörheide, G. (2002). Slow mitochondrial DNA sequence evolution in the Anthozoa (Cnidaria). Molecular Ecology, 11(12), 2475-2487.
- Forsman, Z. H., Hunter, C. L., Fox, G. E., & Wellington, G. M. (2006). Is the ITS region the solution to the “species problem” in corals? Intragenomic variation and alignment permutation in Porites, Siderastrea and outgroup taxa. In Proc 10th Int Coral Reef Symp (Vol. 1, pp. 14-23).
- Fukami, H., Chen, C. A., Chiou, C. Y., & Knowlton, N. (2007). Novel group I introns encoding a putative homing endonuclease in the mitochondrial cox1 gene of scleractinian corals. Journal of molecular evolution, 64(5), 591-600.
- Fukami, H., Chen, C.A., Budd, A.F., Collins, A., Wallace, C., Chuang, Y.Y., Chen, C., Dai, C.F., Iwao, K., Sheppard, C., & Knowlton, N. (2008). Mitochondrial and nuclear genes suggest that stony corals are monophyletic but most families of stony corals are not (Order Scleractinia, Class Anthozoa, Phylum Cnidaria). PloS one, 3(9), e3222.
- Milne Edwards, H. & Haime, J. (1851). Recherches sur les polypiers. Septième mémoire. Monographie des poritides. Annales des sciences naturelles, série 3, Zoologie 16, 21-70, p28.
- Wallace, C. C., Done, B. J. & Muir, P. R. (2012). Revision and catalogue of worldwide staghorn corals Acropora and Isopora (Scleractinia: Acroporidae) in the Museum of Tropical Queensland. Memoirs of the Queensland Museum 57, 1-255.
6.1 Copyrights
- Matson, E. (2008) Permission to publish image sought but not granted because the author is uncontactable. However, usage of the image is within the terms of fair use. http://www.antarctica.gov.au/magazine/2006-2010/issue-15-2008/ocean-acidification/coral-reef-history-books
- Khaled bin Sultan Living Oceans Foundation (2015) Permission to publish image sought but not granted. Awaiting permission.
- Smart Everyday (2014) Permission to publish image sought but not granted. Awaiting permission.
- Ocean Today (2017) Permission is granted to copy, distribute and/or modify this document under the terms of the Creative Commons Attribution-NonCommercial-ShareAlike 3.0 Unported License
- Charles Davis (n.d.) Permission to publish image sought but not granted. Awaiting permission.
- All other photos on this page belong to Copyrights © 2017 Sean Pang. All right reserved, including copy, distribute and/or modify in any way possible.